Life Exponential: Life Exhibits Intelligent Design at Many Levels
Jonathan Wells
Jonathan Wells
A human cell contains two sets of DNA, each consisting of about three billion subunits called “nucleotides.” There are four different nucleotides, and they can be arranged in many different ways, so DNA is quite complex. Most of our DNA, however, must be arranged in a very specific way to provide the information a cell uses to make RNAs and proteins. Mathematician William Dembski has called this “complex specified information.”1
Complexity (such as we see in a pile of autumn leaves) can arise spontaneously from unguided natural processes, but complex specified information cannot. The only known source of complex specified information is an intelligent mind, which can envision a goal and arrange things to actualize that goal — in this case, a living cell. “Because mind or intelligent design is a necessary cause of an information-rich system,” philosopher of science Stephen Meyer wrote in 1998, “the specifically arranged nucleotide sequences — the encoded information — in DNA imply the past action of an intelligent mind, even if such mental agency cannot be directly observed.”2
The Need for Spatial Information
So DNA carries biological information, and that information points to design. But DNA is not the only carrier of information in a living cell. When DNA is transcribed into RNAs, most of those RNAs must be transported to specific locations in the cell before they can function properly. Some RNAs are tagged with sequences called “zipcodes” that specify the “addresses” to which they are to be transported. Like the zipcode on a letter you put in a mailbox, however, an RNA zipcode is meaningless unless it corresponds to a pre-existing address. Like the geographical addresses in a postal system, the cellular destinations of RNAs and proteins must be specified independently of their zipcodes, before they are “mailed.”
Many of those destinations are specified by molecules embedded in cell membranes. Scientists originally thought that proteins could diffuse freely in a membrane, like boats floating on the sea. It is now known, however, that many membrane proteins are arranged in non-random patterns that can be quite stable. Such patterns provide the cell with spatial information that goes beyond the information in DNA.
After fertilization, a frog egg is invisibly divided into regions distinguished by (among other things) spatially localized RNAs just inside the cell membrane in a zone called the “cortex.” In the accompanying drawing, four regions are indicated by different colors. After the nucleus duplicates, the fertilized egg divides into two daughter cells. If the plane of division corresponds to A, each daughter cell inherits not only a nucleus but also portions of all four regions of cortical information. If those two cells are then separated, each can develop into a complete frog. But if the plane of division corresponds to B or C, the daughter cells do not inherit a full set of cortical information, and their development is blocked even if they each contain all the necessary DNA.
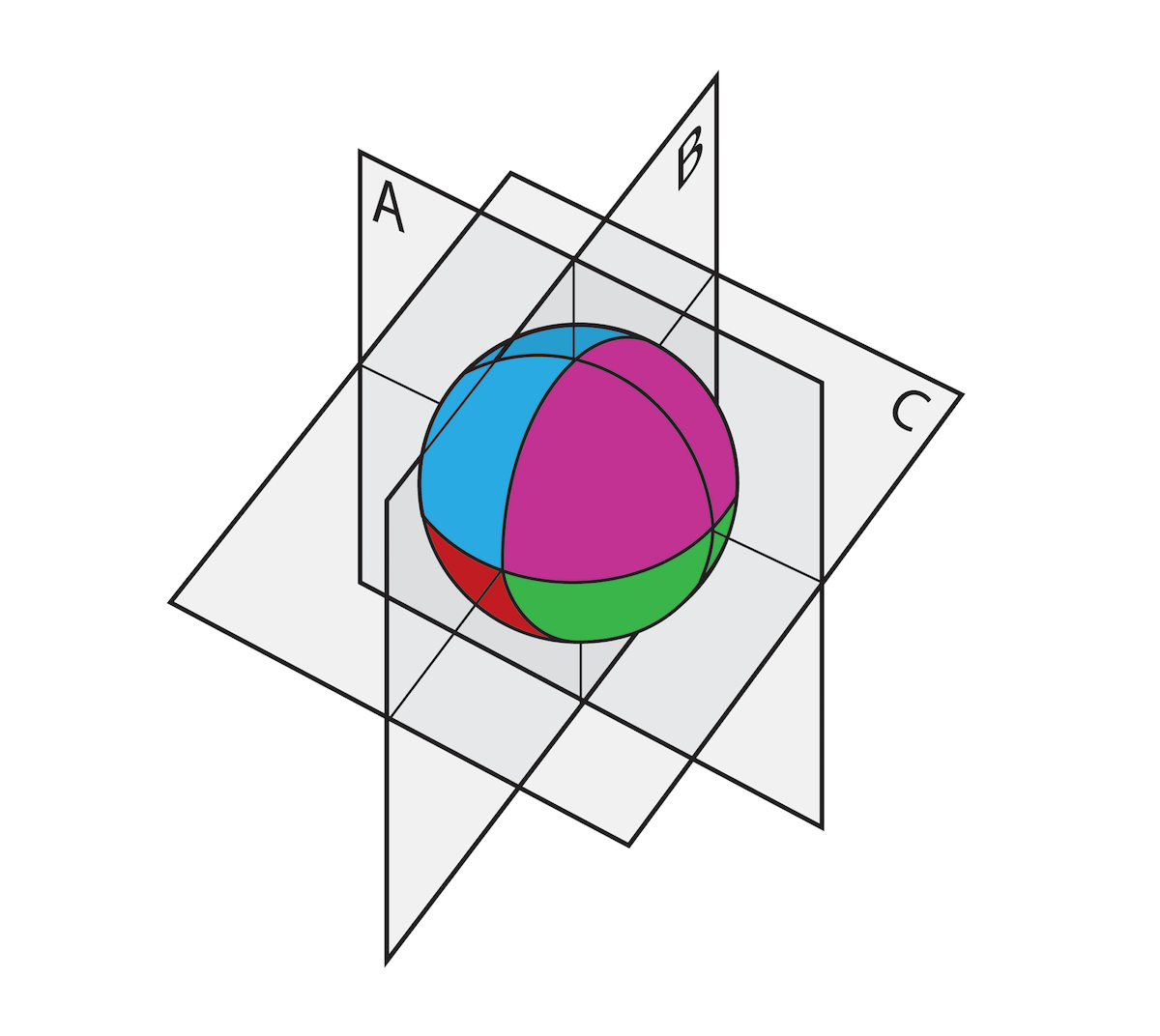
Illustration: Regions of spatial information in a fertilized frog egg.
Other Carriers of Spatial Information
Regional differences in cells and embryos can be specified in other ways besides localization of RNAs in the cortex. Two of those ways have been studied in great detail: the “sugar code” and the “bioelectric code.”
Most proteins in living cells — including those in membranes — are chemically bonded to carbohydrates called “glycans” (from the Greek word for “sweet”). The nucleotides in DNA are linked together end-to-end in a linear molecule, so DNA sequence information is one-dimensional. In living cells, the subunits in proteins (with a few exceptions) are also linked in a linear chain. But glycans can be linked together in complex three-dimensional ways, so their information-carrying capacity exceeds that of DNA and proteins by many orders of magnitude.3
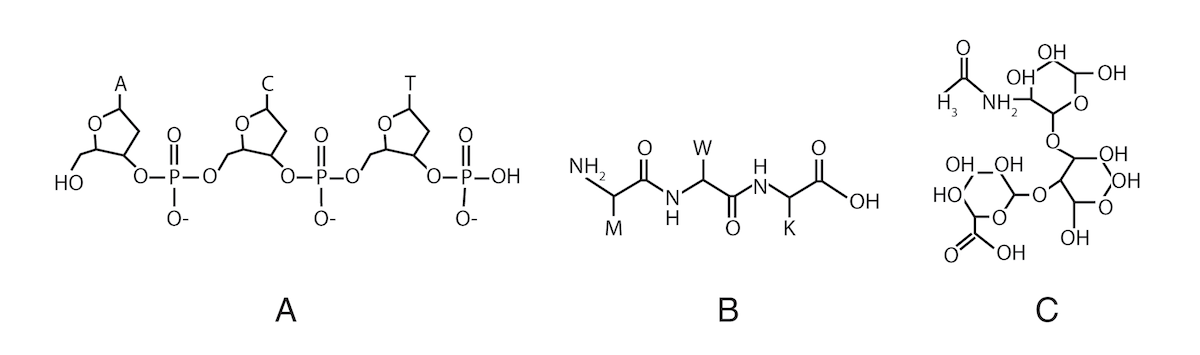
Illustration: Three subunits linked together in DNA (A), a protein (B) and a glycan (C).
The information carried by glycans has been called the “sugar code.”4 The sugar code is “interpreted” by proteins called lectins, which “recognize” specific three-dimensional structures of glycan molecules. Glycans and lectins play an essential role in communication among cells and help to guide cell movements in a developing embryo. Experiments have shown that membrane patterns of glycans change in the course of embryo development.5
In addition to the sugar code, probably all living cells (not just nerve and muscle cells) generate electric fields across their membranes. They do this by pumping charged ions through channels in their membranes, creating a “bioelectric field.” The pattern of membrane channels determines the form of the bioelectric field, and the form of the field changes during embryo development.
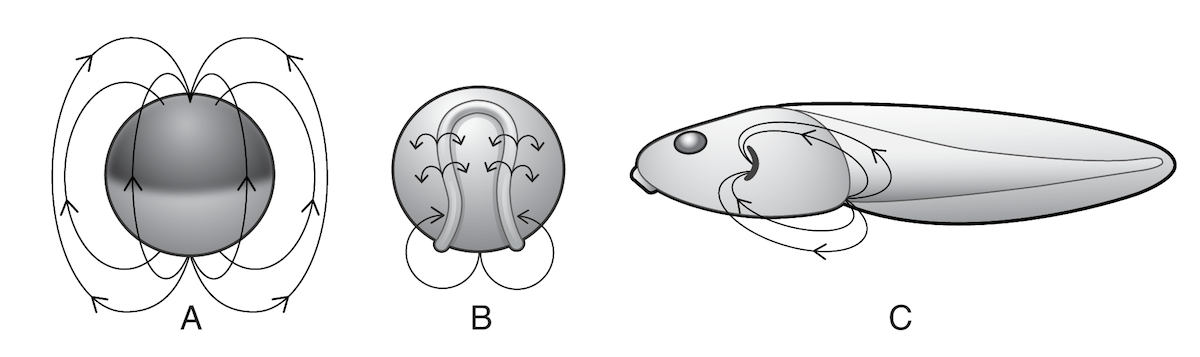
Illustration: Some of the bioelectric fields (shown by arrows) in a developing frog embryo.
Bioelectric fields are correlated with important developmental events. In frog embryos, for example, large ionic currents start flowing out of the sites where the hind limbs will develop long before the limbs actually appear.
Many experiments conducted since the 1980s have confirmed that disrupting bioelectric fields causes disruptions in development. For example, frog embryos normally generate an electric field in the head-to-tail direction. If an artificial field of the same magnitude is applied in the opposite direction, or if ion channels that generate the field are blocked, the result is abnormal head and eye development. The spots where eyes normally form are more highly charged than the surrounding tissue; if the charge is neutralized, the eyes that develop are small or deformed. Sometimes, eyes develop elsewhere on the tadpole’s body, including its tail.5
How do electric fields influence development? In the 1980s, biologists exposed embryonic cells to artificial electric fields of the same strength as ones the cells generate naturally. Some types of cells migrated toward the positive pole, while other types migrated to the negative pole, suggesting that one way bioelectric fields affect embryo development is by directing cell movements.
In 1995, biologists Riyi Shi and Richards Borgens concluded that bioelectric fields “may provide a three dimensional coordinate system” that helps to specify form in embryos.6 In 2013, biologists AiSun Tseng and Michael Levin wrote that such fields may provide “templates of shape,” and that a full understanding of embryo development will probably require cracking the “bioelectric code.”7
The Membrane Code
So localized RNAs in the cortex, glycan patterns on the membrane, and bioelectric fields generated by ion channels in the membrane all carry spatial information. Although individual molecules may be specified by DNA, their three-dimensional patterns are not. Taken together, these patterns constitute a “membrane code” that is independent of DNA sequences.
In 1983, biologist Robert Poyton suggested that biological membranes carry “spatial memory,” the units of which are spatially localized proteins. Poyton wrote: “Realizing that genetic memory is one-dimensional, along a DNA molecule, whereas spatial memory is likely to be two-dimensional, along membrane surfaces, and three-dimensional within the cellular interior, it is probable that spatial memory is more complicated and diverse than genetic memory.”8
In 2004, biologist Thomas Cavalier-Smith wrote that the idea that DNA contains all the information needed to make an organism “is simply false.” According to Cavalier-Smith, membranes provide “chemically specific two-dimensional surfaces with mutually conserved topological relationships in the three spatial dimensions” that play “a key role in the mechanisms that convert the linear information of DNA into the three-dimensional shapes of single cells and multicellular organisms. Animal development creates a complex three-dimensional multicellular organism not by starting from the linear information in DNA… but always starting from an already highly complex three-dimensional unicellular organism, the fertilized egg.”9
So the membrane code carries essential biological information that is independent of DNA sequence information. Yet we often hear that embryo development is directed by a program in DNA. Why?
Beyond DNA
James Watson and Francis Crick’s Nobel Prize-winning discovery of the molecular structure of DNA in 1953 seemed to provide a molecular basis not only for heredity but also for embryo development. Cells replicate their DNA before they divide and (usually) pass on a complete set of their DNA sequences to each of their descendants. Cells then use DNA sequences as templates for the transcription of RNAs, some of which are then translated into proteins.
In the mid 20th century, biology was dominated by neo-Darwinism, a system of thought that combined evolution and genetics and attributed new variations to genetic mutations. An underlying assumption of neo-Darwinism is that evolution and development are due entirely to unguided material processes. After 1953, this materialistic assumption led to the view that “DNA makes RNA makes protein makes us,” which has been called the Central Dogma of molecular biology.
In 1970, molecular biologist (and materialist) Jacques Monod said that with the Central Dogma, “and the understanding of the random physical basis of mutation that molecular biology has also provided, the mechanism of Darwinism is at last securely founded. And man has to understand that he is a mere accident.”9
But the existence of the membrane code shows that the Central Dogma is false. And the materialistic idea that evolution is unguided cannot account for the complex specified information in DNA, much less for the extensive complex specified information in the membrane code. Just as the information in DNA points to design, so does the information beyond DNA.
Notes:
- William A. Dembski, “Intelligent design as a theory of information,” February 20, 1997.
- Stephen C. Meyer, “DNA by design,” Rhetoric and Public Affairs 1 (1998): 519-556.
- Roger A. Laine, “A calculation of all possible oligosaccharide isomers both branched and linear yields 1.05 x 1012 structures for a reducing hexasaccharide,” Glycobiology 4 (1994): 759-767.
- Hans-Joachim Gabius, “Biological information transfer beyond the genetic code: The sugar code,” Naturwissenschaften 87 (2000): 108-121.
- Jonathan Wells, “Membrane patterns carry ontogenetic information that is specified independently of DNA,” Bio-Complexity 2014 (2): 1-28.
- Riyi Shi and Richard B. Borgens, “Three-dimensional gradients of voltage during development of the nervous system as invisible coordinates for the establishment of embryonic pattern,” Developmental Dynamics 202 (1995): 101-114.
- AiSun Tseng and Michael Levin, “Cracking the bioelectric code: Probing endogenous ionic controls of pattern formation,” Communicative and Integrative Biology 6 (2013): e22595
- Robert O. Poyton, “Memory and membranes: The expression of genetic and spatial memory during the assembly of organelle macrocompartments,” Modern Cell Biology 2 (1983): 15-72.
- Thomas Cavalier-Smith, “The membranome and membrane heredity in development and evolution,” pp. 335-351 in Robert P. Hirt and David S. Horner (editors), Organelles, Genomes and Eukaryote Phylogeny (Boca Raton, FL: CRC Press, 2004), 348.
- Jacques Monod, quoted in Horace Freeland Judson, The Eighth Day of Creation: The Makers of the Revolution in Biology (New York: Simon & Schuster, 1979), 217.
Editor’s note: Dr. Wells’s latest book is Zombie Science: More Icons of Evolution. This article first appeared in Salvo 38. It is published here with the permission of Jonathan Wells.
No comments:
Post a Comment